Researchers from the laser-plasma accelerator group have recently published first progress towards the demonstration of the “accelerator” stage of their plasma-modulated plasma accelerator (P-MoPA) scheme, proving its viability for high-energy, high-repetition-rate plasma acceleration.
To efficiently drive a laser-plasma accelerator, the laser pulse duration must be of the order of the size of the plasma wakefield structure (tens of μm), and hence femtosecond-scale pulse durations are typically required. For this reason, Ti:sapphire laser systems have become the standard “workhorse” for the field due to their ability to provide multiple-joule laser pulses with durations on the order of tens of femtoseconds. However, such laser systems have low wall-plug efficiency (~ 0.1%) due to their high quantum defect and so are unable to operate at the kilowatt average-power levels required for the most interesting and promising applications of plasma accelerator technology. The P-MoPA scheme, devised by the laser-plasma accelerator group, unlocks the possibility of using high-repetition-rate, picosecond-long industrial laser systems as drive beams for ultra-compact plasma accelerators.
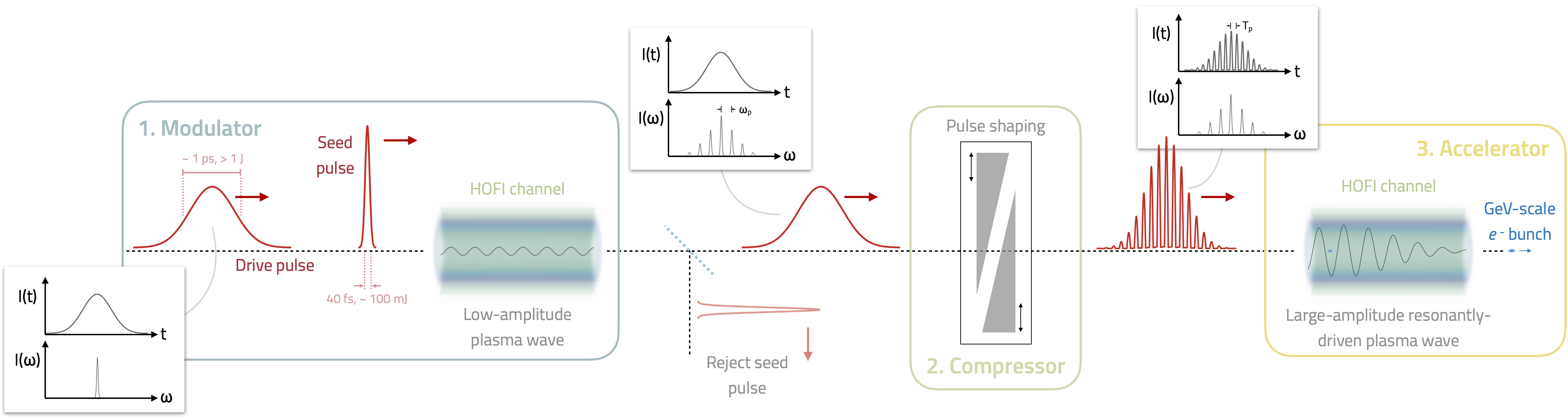
The P-MoPA scheme relies on three stages: (i) the “modulator”, where the picosecond-long, few-joule pulse co-propagates behind a short (40 fs), low energy (≤ 0.1 J) laser pulse in plasma, modifying the long pulse such that it develops spectral “sidebands”. (ii) In the “compressor” stage a specially-designed dispersive system converts the spectral modulation of the long pulse into a temporal modulation, forming a train of short (tens of femtosecond) pulses, each separated by a distance corresponding to the natural frequency of the plasma structure in the “modulator” stage. (iii) The pulse train is used to drive the “accelerator” stage which, when tuned to match the uniform spacing of the P-MoPA pulse train, can resonantly excite a large amplitude wakefield and efficiently accelerate injected electrons.
The paper, titled "Resonant excitation of plasma waves in a plasma channel” and published in Physical Review Research, presents two key advances directly relevant to the P-MoPA scheme. Firstly, it demonstrates that laser pulse trains similar to those produced within the P-MoPA scheme can be guided within plasma channels — a plasma-based “optical fibre” that combats the natural diffraction of a focused laser pulse and extends the interaction distance to the metre-scale. Both the “modulator” and “accelerator” stages of a P-MoPA require the use of plasma channels to maximise their efficiency and enable electron acceleration at the GeV energy-scale — crucial for many applications. Secondly, it was shown that by tuning the on-axis density of the guiding plasma channel to match the pulse spacing, a strong wakefield could be resonantly excited by a pulse train — fundamental to the “accelerator” stage.
The experiment was performed with the Astra-Gemini laser at the Central Laser Facility. Within this experiment it was not possible to generate a pulse train using the full P-MoPA scheme, and hence a “mock-up” pulse train was instead generated via spectral modulation of a few-joule chirped, picosecond-long Ti:sapphire laser pulse. The resonant response of the plasma to the pulse train was measured by detecting shifts in the spectrum of the pulse train after it had interacted with the plasma. A strong red-shift of the spectrum indicated that the pulse had transferred a significant fraction of its energy to the plasma, only possible when a large amplitude wakefield is being driven. The experimentally-measured red-shift was found to agree well with numerical modelling of the interaction, indicating wakefield amplitudes of 3-10 GV/m being excited within the plasma, sufficient for GeV-scale energy gain within a distance of only 100 mm — orders of magnitude smaller than required in conventional radio-frequency accelerators, whose accelerating gradients are typically limited to < 0.1 GV/m.
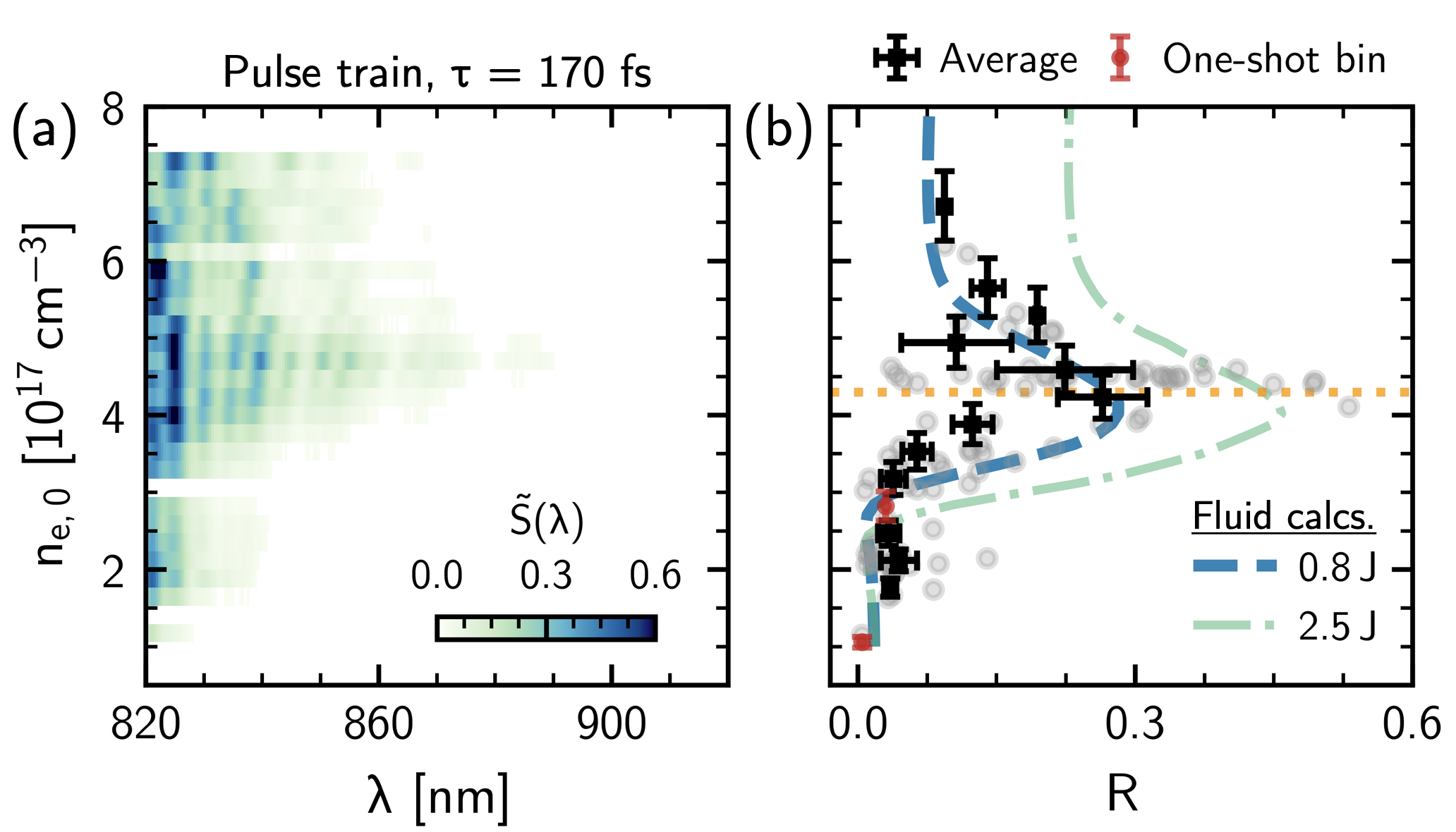
The research represents a significant step forward in the development of compact, efficient, and practical laser-driven particle accelerators. By addressing the limitations of current “work-horse” laser technologies and demonstrating the feasibility of the P-MoPA approach, the study opens up new possibilities for the application of laser-driven accelerators across various scientific and industrial domains. In medical applications, for example, compact and efficient laser-driven accelerators could provide more precise and less invasive treatments for cancer patients through advanced radiation therapy techniques. In materials science, these accelerators could be used to probe the properties of materials at unprecedented scales, leading to new discoveries and innovations. Additionally, in fundamental physics, high-energy particle beams generated by laser-driven accelerators could facilitate experiments that explore the fundamental properties of matter and the universe.
For further details, the full research paper is available here.