A new study led by Professor Shilei Zhang from ShanghaiTech University, involving the University of Oxford and Diamond Light Source, has uncovered a groundbreaking phenomenon in the dynamics of magnetic skyrmions, which are topologically protected spin textures with particle-like properties. Traditionally, skyrmions have been understood to move linearly in response to external stimuli. However, the research team's innovative approach using a linear temperature gradient has revealed a new, continuous rotational motion within skyrmion crystallites, driven by a novel chiral lattice torque.
Skyrmion crystallites as rigid entities
Magnetic skyrmions are known for their potential as information carriers in spintronics due to their stability, low-energy transport cost and nanoscale size. As for conventional magnetic information carriers, speed is key so the study of their dynamics is an important task on their way to device applications. In certain chiral magnets, skyrmions condense into a skyrmion lattice – a two-dimensional, closely-packed hexagonal arrangement. Unlike isolated skyrmions, this condensed skyrmion lattice exhibits a unique rigidity, meaning that the packed skyrmion structure resists to be broken up into its constituent parts. Under inhomogeneous driving forces, however, the strain on the skyrmion lattice becomes large enough to break the perfect lattice up into finite-size skyrmion crystallites. The team's experimental work demonstrated that when subjected to a linear temperature gradient, these skyrmion crystallites do not merely translate; they also rotate, a motion analogous to a rolling ball or drifting sheets of ice in the ocean, combining both translational and rotational dynamics.
Experimental breakthrough with resonant elastic X-ray scattering
Utilising time- and spatially-resolved resonant elastic X-ray scattering (REXS) at beamline I10, the researchers observed this rotational behaviour in the insulating chiral magnet Cu2OSeO3. Their experiments provided direct evidence of the chiral lattice torque's role in driving the skyrmion crystallites' rolling motion. The skyrmion crystallites displayed a uniform rotational chirality, which could be reversed by flipping the direction of the applied magnetic field, confirming the theoretical predictions.
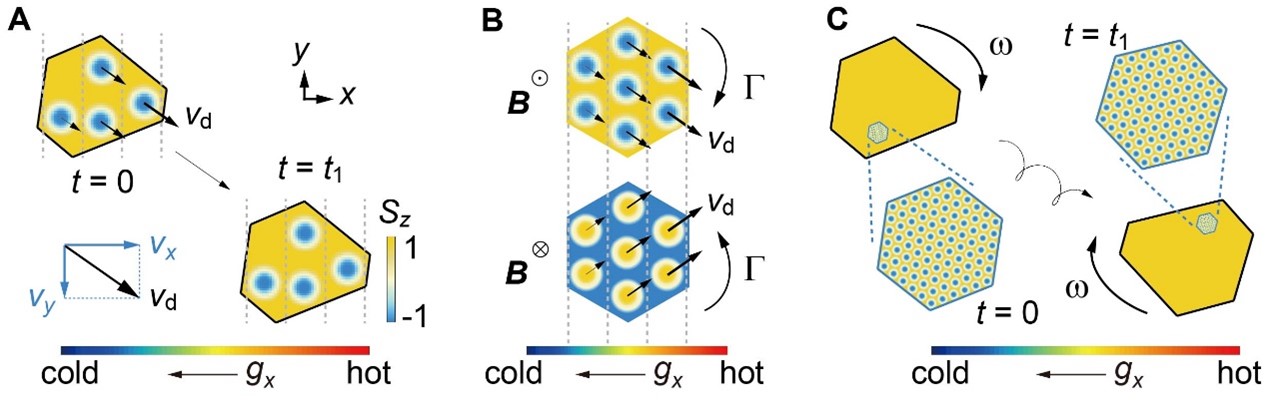
Implications and future directions
This discovery not only challenges the conventional understanding of skyrmion dynamics but also introduces a new degree of freedom for manipulating these quasi-particles, potentially leading to novel applications in spintronics and data storage. The ability to control both the translational and rotational motions of skyrmions opens up exciting possibilities for future research and technology development.
Professor Shilei Zhang, the lead author, expressed his excitement about the findings: 'Discovering that skyrmion crystallites roll like tiny wheels under a simple temperature gradient opens up a whole new way of thinking about their manipulation.' He further stated that these rotational dynamics could be a game changer in how we design future spintronic devices.
For more information on the subject matter, please contact either Professor Shilei Zhang (ShanghaiTech University): shilei.zhang@shanghaitech.edu.cn, Professor Gerrit van der Laan (Diamond Light Source): gerrit.vanderlaan@diamond.ac.uk, or Professor Thorsten Hesjedal (Oxford Physics): Thorsten.Hesjedal@physics.ox.ac.uk.
To find out more about the I10 beamline, or to discuss potential applications, please contact Principal Beamline Scientist Paul Steadman: paul.steadman@diamond.ac.uk