In many of today's most interesting materials strong interactions prevail upon the magnetic moments, the electrons and the underlying crystal structure, often forming strong links between these different aspects of the system. Such materials can exhibit exciting physical phenomena whose description requires new quantum mechanical models to be developed. Examples include superconductors, magnets, topological insulators, and multiferroics.
Several research groups within the Department of Physics are actively studying quantum matter, exposing a variety of materials to different experimental techniques in order to gain a better understanding of quantum theories of matter and to exploit the findings for the next generation of functional materials and devices. We work collaboratively with groups in theoretical physics, chemistry, materials science and experimental physics both within Oxford and beyond.
Magnetism
Forcing magnetic moments to lie in chains, planes, triangles and other non-cubic arrangements strengthens some of the quantum mechanical interactions between the moments while hindering others. In this way, it is possible to produce magnetic phases and novel behaviour not seen in higher symmetry systems. By making measurements on low-dimensional magnetic materials, we experimentally explore the mechanisms responsible for these exotic properties, map out new magnetic states and evolve current models of quantum magnetism.
Materials development
Our materials development research groups are consistently cultivating fresh compounds and new sample configurations to better understand the science behind many different physical phenomena. By altering the composition of materials the crystal growth unit adjusts the electronic correlations that are responsible for magnetism and superconductivity, while the thin film research group controls the lattice parameters of interesting systems, tuning their properties without the need for doping.
Multiferroics
Multiferroics can be described as materials in which there are couplings between different types of ordering, including magnetic, charge, and elastic orders. The linkages mean that the properties of these systems are highly susceptible to multiple external perturbations, for example we might access control of the magnetic order by dropping a voltage across the sample, or actuate a strain within the crystal by applying a magnetic field. Clearly abilities like these are ripe for technological application, but first we need to understand why these couplings develop and how to foster them so that we can find a recipe for creating multiferroics with made-to-measure properties. We do this by applying the full range of our experimental capabilities to investigate the ordered phases found within these fascinating materials.
Spin systems
Spintronics utilises the intrinsic spin of electrons to create state of the art solid state devices that out-perform, or provide services not offered by, traditional technologies based on the electronic charge. The quantum phenomena of superposition and entanglement are also exploited to develop a new type of quantum logic that has the potential to revolutionise computational processes as we know them.
Superconductivity
Superconductivity, the phenomenon of zero electrical resistance and magnetic field expulsion, which occurs below a critical temperature in certain materials, has a huge potential for technological applications. Electrons in superconductors are known to join together in pairs in order to gain immunity from scattering, but exactly how this occurs in many materials has yet to be established. At present, superconducting critical temperatures are typically very low, less than 140 Kelvin (-133 Celsius), and even those materials with the highest critical temperatures have physical properties that makes them challenging to exploit on an industrial scale. A more complete understanding of the superconducting state will result in the design of superconducting materials and devices with the properties required for mass technological exploitation.
We pursue an number of avenues to this end. The low temperature properties of novel superconducting materials, including copper-oxide compounds, pnictides, and organic superconductors, are investigated using high magnetic fields, neutron scattering, muon spin rotation and thermal transport techniques.
Experimental techniques
We use a variety of techniques including electron spin resonance to excite the spins inside a material and learn about the local environment; the application of high magnetic fields to reveal the complex behaviour found in quantum materials; muon spin rotation where the muon behaves as a 'microscopic magnetometer' to investigate both static and dynamic effects; and x-ray and neutron scattering to understand the structures and dynamics present in quantum materials at the atomic level.
Materials of interest
Complex oxides: high temperature superconductivity, quantum magnetism and multiferroic behaviour can all be found in the complex oxide family of materials. These intricate crystalline arrangements of oxygen and other, typically metallic, ions support strong and highly directional electronic interactions which bolster the exotic phases of matter they display.
Iron-based superconductors: our work concentrates on studying these new surprising superconductors using neutrons, muons and fermiological techniques working closely with a group in inorganic chemistry.
Organic and molecular systems: we study purely organic ferromagnets, molecular magnets built up by joining up transition metal ions with molecular linkers, spin liquids and molecular superconductors. These materials provide wonderful opportunities to study electronic, magnetic and superconducting behaviour in a chemically-controlled environment.
Condensed Matter Physics Graduate Prospectus
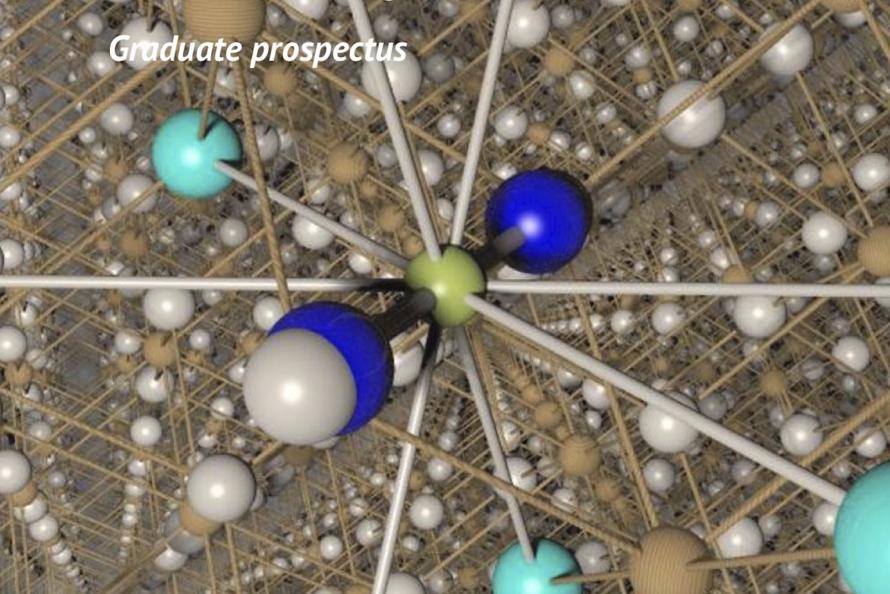
Experimental facilities
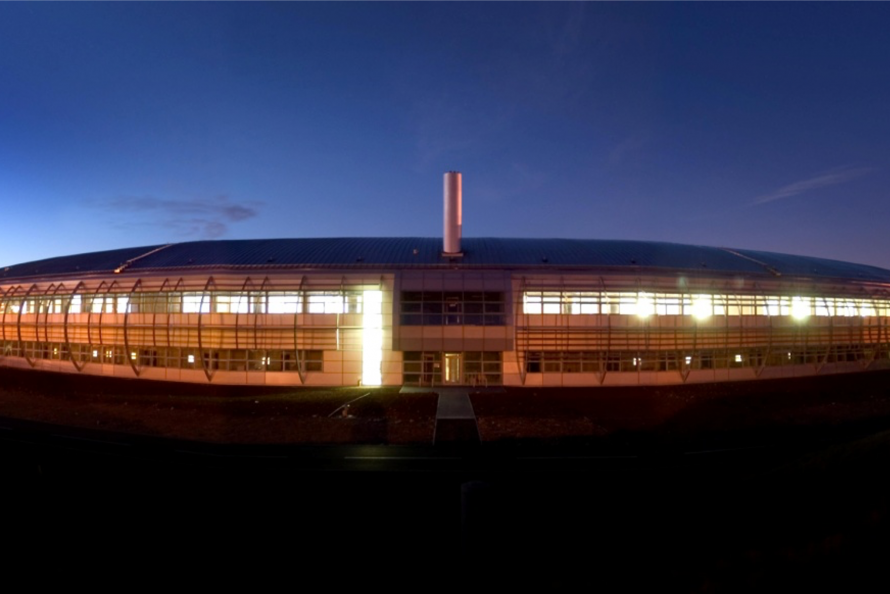
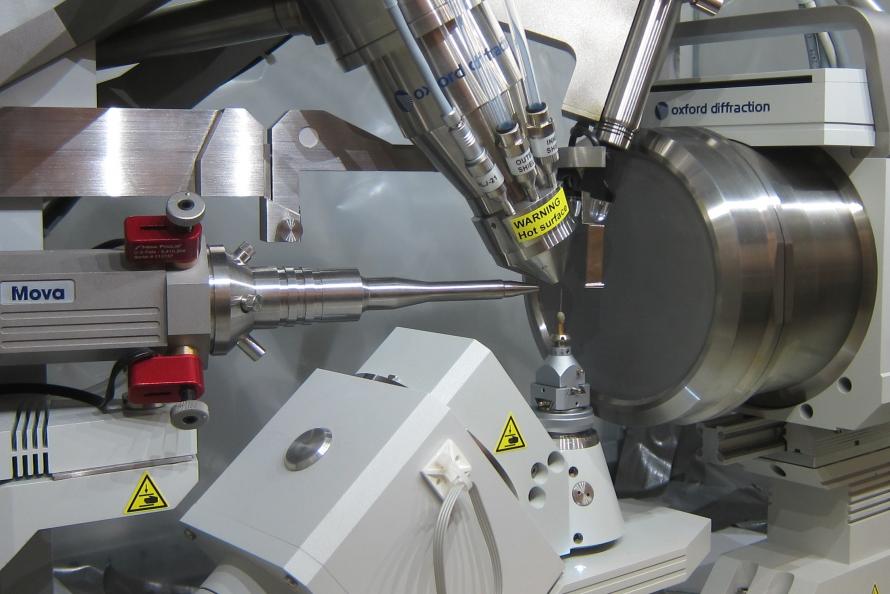