Galactic explosions and their fallout
Supervisors: Katherine Blundell
Nova explosions occur much more frequently than supernova events and arise as the result of a thermonuclear runaway on the surface of a white dwarf. The recent discovery of jets being ejected at the onset of a nova explosion, which have speeds of a few thousand km/s, suggests an important means by which the inter-stellar medium can be enriched by the products of nucleosynthesis that take place on the surface of the white dwarf. The goal is to investigate the mechanisms by which these processes take place, and the efficacy of enrichment of the ISM by jets from nova explosions, using time-lapse data from the Global Jet Watch (PI K Blundell; www.GlobalJetWatch.net).
Circumbinary discs and planets
Supervisors: Katherine Blundell
In recent years the existence and significance of circumbinary discs, orbiting outside of pairs of binary stars in orbit around one another, has emerged. Not only are these purported to have significant dynamical back-reactions on their inner binary stars (and hence their evolution) and in the development of nova explosions but they are in some cases likely to be the breeding ground of Tatooine-like circumbinary planets. The goal is to explore and understand the nature of binary star systems that host such circumbinary structures, using data from the Global Jet Watch telescopes (PI K Blundell; www.GlobalJetWatch.net).
Type Ia Supernova cosmology with the Rubin Observatory’s Legacy of Space and Time
Supervisors: Maria Vincenzi, Stephen Smartt
It's an exciting time for cosmology. Over the past 12 months, numerous independent cosmological studies have been published, each employing very different techniques (Type Ia Supernovae, Baryonic Acoustic Oscillations, Cosmic Microwave Background). Remarkably, all these findings seem to point to the same conclusion: the properties of Dark Energy are more complex than what we have previously understood.
Type Ia supernovae (SNe Ia) are among the most well-established tools in modern cosmology, with dozens of successful cosmological experiments conducted over the past three decades. The Vera C. Rubin Observatory's Legacy Survey of Space and Time (LSST), set to begin in January 2025, is expected to significantly transform the field. In just the first few months of observations, LSST will discover more SNe Ia than observed since the field began, thus revolutionizing SN Ia cosmological measurements and transient astronomy as a whole.
Our team is leading the preparation for the beginning of LSST and will be heavily involved in the analysis of the first LSST transient data. We work on a wide range of projects (both observations oriented and simulations oriented), revolving around the development and testing of transient discovery and classification pipelines, optimisation of LSST spectroscopic follow-up programs, and the design of new strategies to compile cosmological samples using LSST supernova data.
In collaboration with UK scientists, we are also leading the TiDES spectroscopic follow-up program, which will use the 4MOST spectrograph to follow up on tens of thousands of LSST transients and their host galaxies, providing crucial insights into their properties and redshifts.
The DPhil project will focus on preparing for future SN Ia cosmological measurements using LSST and TiDES data, with an emphasis on addressing key sources of uncertainty that impact these measurements.
Understanding the extremes of the transient Universe
Supervisors: Stephen Smartt, Maria Vincenzi
Over the last number of years a number of explosive transients have been discovered with uncertain physical nature. These include fast x-ray transients, luminous fast blue optical transients and infrared transients associated with long gamma ray bursts. They may be tidal disruption events from intermediate mass black holes, black hole forming supernovae, and kilonovae from merging neutron stars.
The Rubin Observatory will begin operations in late 2025, providing the deepest time domain survey of the sky ever undertaken. Combing the optical discoveries with high energy and radio surveys has the potential to unveil the nature of these source and may reveal new populations. We use machine learning methods to discover and classify these transients in ongoing sky surveys. By combining the nightly data flow from LSST with higher energy (gamma to x-ray) emission and fast spectroscopic classification from the new spectrometer at the European Souther Observatory, we will discover large numbers of these extreme transients and fit physical models to their emission.
The astrophysics of Ultra-Long-Period-Transients.
Supervisors: Aris Karastergiou, Kaustubh Rajwade
Compact objects such as neutron stars provide unique experiments into fundamental physics. These stars boast extraordinarily high nuclear densities and magnetic fields inferred to be over a billion times stronger than humans have ever generated. Our current understanding of neutron stars suggests that they are born with short spin periods (tens to hundreds of milliseconds) and slow down with time, as a consequence of their strong and misaligned magnetic field. Some of them emit radio waves, powered by the rotational energy, as they age but the emission eventually ceases as they slow down to large spin periods (> 60 seconds). This idea has been challenged recently with the discovery of an unanticipated class of radio-emitting compact objects with ultra-long spin periods (called Ultra-Long Period Transients (ULPTs)). Some of these objects exhibit neutron star properties. Furthermore, this new population could hold the key to explaining another tantalizing astrophysical phenomenon. Fast Radio Bursts (FRBs) are luminous radio flashes that carry as much energy in a millisecond as the Sun produces in three days, making them detectable at cosmological distances, and useful for solving important questions in Cosmology. The recent detection of luminous radio bursts from a Galactic neutron star already suggests a physical link between FRBs and neutron stars. In this project, the student will address key questions on the astrophysics of ULPTs, namely
What are the possible pathways of neutron star evolution leading to long-period sources?
and
What powers the radio emission in ULPTs, and can that explain FRBs?
The student will play a key role in a comprehensive search and a subsequent monitoring campaign of a population of long-period neutron stars using radio images from the Low Frequency Array (LOFAR), a premier radio telescope operating at low radio frequencies. Furthermore, this project will leverage the UK's radio astronomical infrastructure (Lovell telescope + e-MERLIN array) to carry out a detailed radio study of long-period neutron stars and a sample of FRBs. The project provides ample opportunity for the student to explore new directions, develop new search algorithms and test new candidate classification schemes, harnessing the power of high-performance computing and artificial intelligence.
Are Fast Radio Bursts emitted by Neutron Stars?
Supervisors: Aris Karastergiou, Kaustubh Rajwade
Fast Radio Bursts (FRBs) are a cosmic phenomenon whose origins have remained uncertain for more than a decade. Owing to their large radio brightness and extreme distances, these short-duration bursts signify processes with the highest energy densities in the Universe and are potential probes to solve key open questions in Cosmology. Recent observations have suggested that neutron stars, remnant cores of stars that die in a brilliant supernova explosion, could explain some of the phenomena seen in FRBs. Understanding the source of FRBs will provide an advancement of physics in extreme conditions and enable studies of matter and plasma in extreme gravity and magnetic fields, experiments untenable in laboratories on Earth. Understanding the connection between neutron stars and FRBs has been a challenge. While FRB-like bursts have been observed from a Galactic neutron star, there is still a disparity between the luminosities of FRBs and typical radio pulses from neutron stars (i.e pulsars). Most studies so far have only focussed on isolated sources that lack statistical quantification. A systematic comparative study of a large sample of FRBs and radio pulses from neutron stars is a crucial step toward establishing the connection between neutron stars and FRBs. This has not been possible until recently, owing to a small number of published FRBs and a lack of a large sample of single pulses from one radio telescope.
Now using two extensive datasets of FRBs and individual pulses from pulsars, this project will pioneer a comprehensive population study of FRBs and radio pulses from neutron stars to establish the extent of the connection between these two types of objects. We co-lead the Thousand Pulsar Array project (TPA) on MeerKAT, which has collected a dataset of more than a million single pulses from neutron stars (including giant pulses and bright pulses from highly magnetized neutron stars) taken with the MeerKAT telescope, providing the best possible reference for comparison. The CHIME/FRB collaboration has recently published an entire catalog of more than 500 FRBs with high-time resolution and polarisation data for more than 120 FRBs in the largest uniform dataset on FRBs yet. Combining this dataset with the one from the TPA project, this project will attempt to answer the following fundamental questions:
Can we explain FRB phenomenology as radio bursts from neutron stars?
and
Is there a continuum of coherent radio emitters from neutron stars to FRB?
The project offers an exploration of the state of the art of neutron star astrophysics, and the possibility of using high performance computing and artificial intelligence techniques.
Understanding the population of radio pulsars using MeerKAT
Supervisors: Aris Karastergiou
Using pulsars for experiments in fundamental physics relies more and more on a detailed understanding of the physics of neutron stars. Despite many decades of progress in the field, there remain open fundamental questions, such as the birth periods of pulsars, the mechanism by which they evolve with time, and the radio emission mechanism.
The Thousand Pulsar Array survey with the MeerKAT telescope in South Africa is providing a paradigm setting dataset of a very large population of radio pulsars, observed monthly. Time-domain and imaging data are both recorded and analysed. In addition to revealing how a large population of pulsars evolve with time, monthly monitoring allows us to separate those effects that are intrinsic to the star from those related to propagation of the radiation as it travels through the intervening media. The student will work within an international collaboration (www.meertime.org) to address key questions in pulsar population studies, relating to understanding the cold and dense nuclear matter in neutron star interiors, the plasma physics processes the occur in pulsar magnetospheres, the properties of the ionized and magnetized interstellar medium, and the birth and evolution of neutron stars. Understanding the neutron star population has far reaching impact in astrophysics and Gravitational Wave astronomy.
The Next Phase of Technosignature Searches: Streaming Processing for Transients and SETI
Supervisors: Andrew Siemion, Alex Pollak, Joe Bright
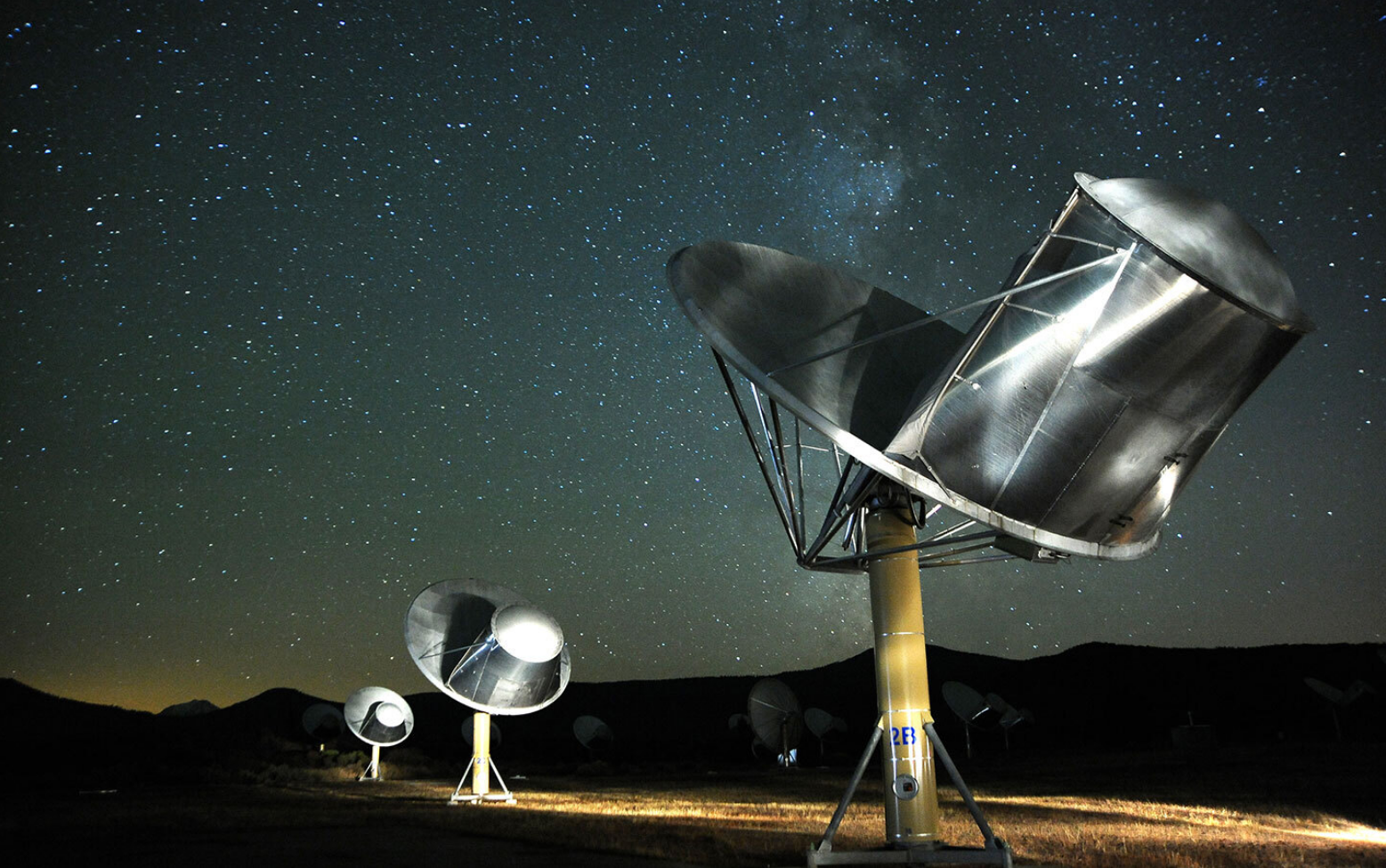
Breakthrough Listen, headquartered in the Department of Physics since 2023, is humanity’s most comprehensive search for technosignatures (indicators of technology as a proxy for extraterrestrial intelligence). The Listen team employs cutting-edge digital hardware at telescopes across the world and in space, developing new algorithms and approaches to ingest and process enormous streams of data.
With instruments such as the Vera C. Rubin Observatory soon starting their surveys of the sky, and pathfinders and precursors to the Square Kilometre Array telescope already producing outstanding science, the time is ripe to harness the tools of modern astronomy to expand technosignature searches into their next phase.
One SKA pathfinder, the Allen Telescope Array, was recently upgraded to support streaming digital signal processing as part of a collaboration with NVIDIA. Using NVIDIA Holoscan, a cutting-edge GPU-accelerated digital signal processing pipeline, SETI Institute and NVIDIA researchers, in collaboration with the Listen team, demonstrated real-time machine learning processing for the detection of fast radio bursts.
The incoming student will be expected to build on the prototype system to deploy a full technosignature and transient detection pipeline at the ATA, and from there to expand deployment to other facilities with existing SETI Institute and Listen backend processing instruments. Real time processing will enable data to be analysed at the native frequency and time resolution delivered from the telescope (impossible in archival data because of time and frequency averaging used to make data volumes manageable). Streaming processing will enable subsets of data on interesting signals to be archived, as well as triggering other facilities to undertake real-time follow-up.
The student will be expected to have a background in high performance computing, digital signal processing, and / or GPU programming.
Comprehensive High-Frequency Survey of the Galactic Center for Technosignatures and Pulsars
Supervisors: Andrew Siemion, Vishal Gajjar, Joe Bright
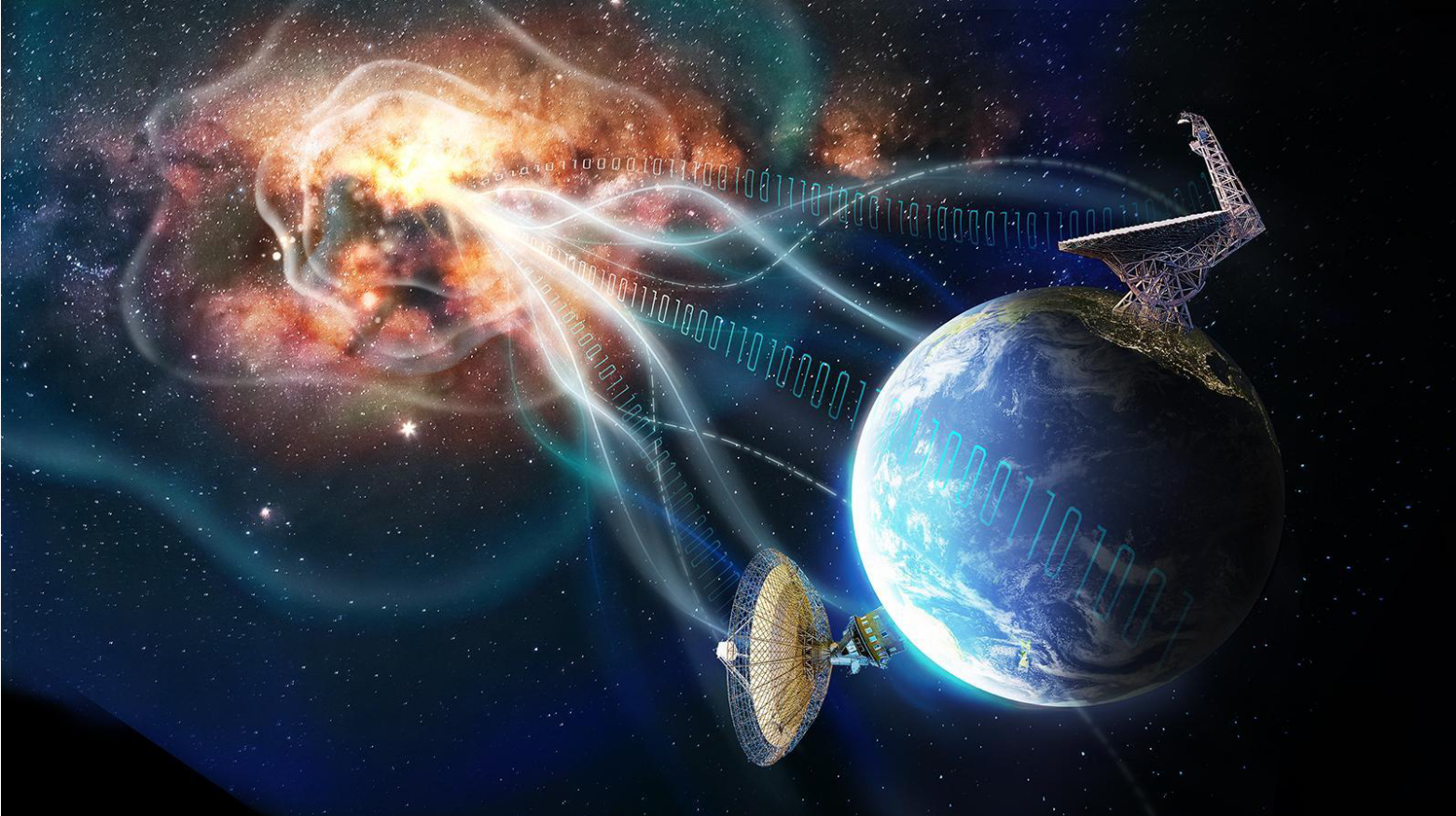
The Galactic Center (GC) region of the Milky Way is one of the most dynamic areas, hosting a vast array of diverse and fascinating astrophysical objects. The Breakthrough Listen project — the most comprehensive initiative aimed at searching for evidence of technologically-advanced life (technosignatures) — is undertaking one of the deepest surveys of the GC, covering frequencies from 1 to 93 GHz with nearly 600 hours of observations. The line of sight toward the GC includes the highest concentration of potentially habitable planets within the Milky Way, making it an ideal direction for technosignature searches. Additionally, the GC region likely hosts numerous radio pulsars and magnetars, with a significant portion orbiting the central supermassive black hole (SMBH). Detecting a pulsar in close orbit around the SMBH would allow for the most precise tests of general relativity to date.
The incoming DPhil student will lead observations using two of the world’s largest single-dish telescopes: the Green Bank Telescope (GBT) in the USA and the Parkes “Murriyang” Telescope in Australia. The student will design and deploy novel AI-based signal search algorithms to identify various types of radio technosignatures (Paper I). Additionally, the student will conduct some of the first high-frequency surveys of the GC for radio pulsars, utilising the 12–15.5 GHz Ku receiver and the 18–27.5 GHz multibeam KFPA receiver on the GBT utilising advanced AI-based pulsar search techniques (Paper II).
Suggested Reading:
Gajjar et al. 2021, The Astronomical Journal, Volume 162, Page 33
Nonthermal particle acceleration in extreme plasmas around black holes
Supervisors: Dmitri Uzdensky
Accreting supermassive black holes (SMBHs) residing in active galactic nuclei (AGN) at the centres of many galaxies, including the Milky Way, are arguably the most fascinating and enigmatic objects in the Universe. These black holes, and the powerful relativistic jets emanating from them, often exhibit spectacular, violent phenomena, such as bright high-energy flares with nonthermal X-ray and gamma-ray spectra. They are also the most likely generators of energetic non-electromagnetic observational "messengers": extremely relativistic cosmic rays and ultra-high-energy neutrinos. All these observational signals indicate that BH environments are very efficient relativistic-particle accelerators. Understanding how these cosmic accelerators work is an outstanding problem in high-energy astrophysics. Nonthermal particle acceleration is believed to be a natural product of nonlinear collective kinetic plasma processes, such as magnetic reconnection, shocks, and magnetised turbulence. How these processes operate under the extreme physical conditions in the exotic relativistic plasmas of accreting black holes is not well understood because the underlying physics is much richer than in more traditional plasmas (like ones in the solar system): special- and general-relativistic effects, strong interaction of the plasma with radiation, and quantum-electrodynamic effects such as pair creation all come into play in nontrivial ways. Elucidating the complex interplay of these effects with collective plasma processes and, consequently, their impact on particle acceleration and radiation presents an exciting theoretical challenge and is a vibrant frontier of modern plasma astrophysics research. This project will explore these fundamental plasma-theoretical questions and their observational implications through a combination of analytical and computational methods, with the balance between theory and computation to be decided based on the student's preferences.
Generating and sustaining radio filaments in astrophysical plasmas
Supervisors: Archie Bott, Alexander Shekochihin
One of the most intriguing findings from recent high-resolution astronomical observations of radio emission in astrophysical objects, such as galaxy clusters (as well as our own Galaxy), is the presence of collimated filaments of plasma [1-6]. These structures, which can extend from tens [2] to hundreds [4] of kiloparsecs, pose a significant theoretical challenge: how they are formed and persist are open questions. If the evolution of these plasma filaments were modelled accurately by hydrodynamics, they would quickly break up due to the Kelvin-Helmholtz and Rayleigh-Taylor instabilities. Yet they are there, and they shine with synchrotron radiation---which suggests that both the fluid instabilities are suppressed, and also that the filaments are capable of channelling high-energy cosmic rays, thought to produce the observed radio emission. A plausible hypothesis is that these filaments are threaded by magnetic fields with energy densities comparable to the thermal energy density of the plasma. However, the mechanism by which such strong magnetic fields could be generated in the low-density, high-temperature plasma typical of intergalactic space is a mystery. In this DPhil project, we will investigate how (or whether) strong compression and shearing of intergalactic plasma can lead to emergence of a "two-phase" intracluster medium: strongly and weakly magnetised, with a particular focus on the nonlinear evolution of the so-called mirror instability [7-9]. The research will involve analytical work, as well as fluid and kinetic.
Background Reading:
- R. J. van Weeran et al., Space Sci. Rev. 215, 16 (2019)
- M. Brienza et al., Nature Astron. 5, 1261 (2021)
- L. Rudnick et al., Astrophys. J. 935, 168 (2022)
- K. Rajpurohit et al., Astrophys. J. Lett. 927, L80 (2022)
- F. Yusef-Zadeh et al., Astrophys. J. Lett. 939, L21 (2022)
- E. Churazov et al., Astron. Astrophys. 686, A14 (2024)
- M. W. Kunz et al., Phys. Rev. Lett. 112, 205003 (2014)
- F. Rincon et al., MNRAS 447, L45 (2015)
- S. Melville et al., MNRAS 459, 2701 (2016)
Magnetised plasma turbulence: from laser lab to galaxy clusters
Supervisors: Gianluca Gregori, Archie Bott, Alexander Schekochihin
There are a number of possibilities within this project to design, take part in, and theorise about laboratory experiments employing laser-produced plasmas to model astrophysical phenomena and basic, fundamental physical processes in turbulent plasmas. Recent examples of our work in this field include turbulent generation of magnetic fields ("dynamo") [1,2], supersonic turbulence mimicking star-forming molecular clouds [3], diffusion and acceleration of particles by turbulence [4,5], suppression of thermal conduction in galaxy-cluster-like plasmas [6]. Our group has access to several laser facilities (including the National Ignition Facility, the largest laser system in the world). Students will also have access to a laser laboratory on campus, where initial experiments can be fielded. Depending on the student's inclinations, it is also possible to pursue a project focused on theory and/or numerical modelling of plasma phenomena in astrophysical and laboratory-astrophysical environments.
References:
- P. Tzeferacos et al., "Laboratory evidence of dynamo amplification of magnetic fields in a turbulent plasma," Nature Comm. 9, 591 (2018) (2019 APS Dawson Prize)
- A. F. A. Bott et al., "Time-resolved fast turbulent dynamo in a laser plasma," PNAS 118, e2015729118 (2021) (2020 EPS PhD Award in Plasma Physics)
- T. G. White et al.,"Supersonic plasma turbulence in the laboratory," Nature Comm. 10, 1758 (2019)
- A. F. A. Bott et al., "Proton imaging of stochastic magnetic fields," J. Plasma Phys. 83, 905830614 (2017)
- L. E. Chen et al., "Transport of high-energy charged particles through spatially intermittent turbulent magnetic fields," Astrophys. J. 892, 114 (2020)
- J. Meinecke et al., "Strong suppression of heat conduction in a laboratory analogue of galaxy-cluster turbulent plasma," Science Adv. 8, eabj6799 (2022)
Microphysics of Gamma-ray Bursts
Supervisors: Gianluca Gregori, Subir Sarkar, Dmitri Uzdensky
Gamma-ray bursts (GRBs) are among the most energetic events in the Universe. They occur at cosmological distances and are the result of the collapse of massive stars or neutron stars mergers, with emission of relativistic "fireballs" of electron-positron pairs. From astrophysical observations, a wealth of information has been gleaned about the mechanism that leads to such strong emission of radiation, with leading models predicting that this is due to the disruption of the beam as it blasts through the surrounding plasma. This produces shocks and hydromagnetic turbulence that generate synchrotron emission, potentially accelerating to ultra-high energies the protons which are observed on Earth as cosmic rays. However, there is no direct evidence of the generation of either magnetic fields or cosmic rays by GRBs. Estimates are often based on crude energy equipartition arguments or idealized numerical simulations that struggle to capture the extreme plasma conditions. We propose to address this lacuna by conducting laboratory experiments at accelerator facilities to mimic the jet propagation through its surrounding plasma. Our intial work [1] has demonstrated that we can create enormous beams of electron-positron plasmas that have properties very similar to GRB Fireballs. We are now planning new experiments at CERN as well as at Laboratori Nazionali Frascati (INFN, Italy) to fully characterize the interaction of these beams with a surrounding (ambient) plasma. Such experiments will enable in-situ measurement of the plasma properties, with exquisite details that cannot be achieved elsewhere. The experiments also complement numerical simulations by providing long measurement times extending into the non-linear regime where numerical simulations are not possible today. The proposed experiments will study fundamental physics processes, unveil the microphysics of GRBs, and provide a new window in high energy astrophysics using novel Earth-based laboratory tools.
Background Reading:
- C. D. Arrowsmith et al., "Laboratory realisation of relativistic pair-plasma beams," Nature Comm. 15, 5029 (2024)