Harnessing topological nano-whirls to enable brain-like computing
Supervisor: Hariom Jani
There are 2 Royal Society-funded DPhil scholarships available to support this project. Students will have the opportunity to be co-supervised by Paolo G. Radaelli.
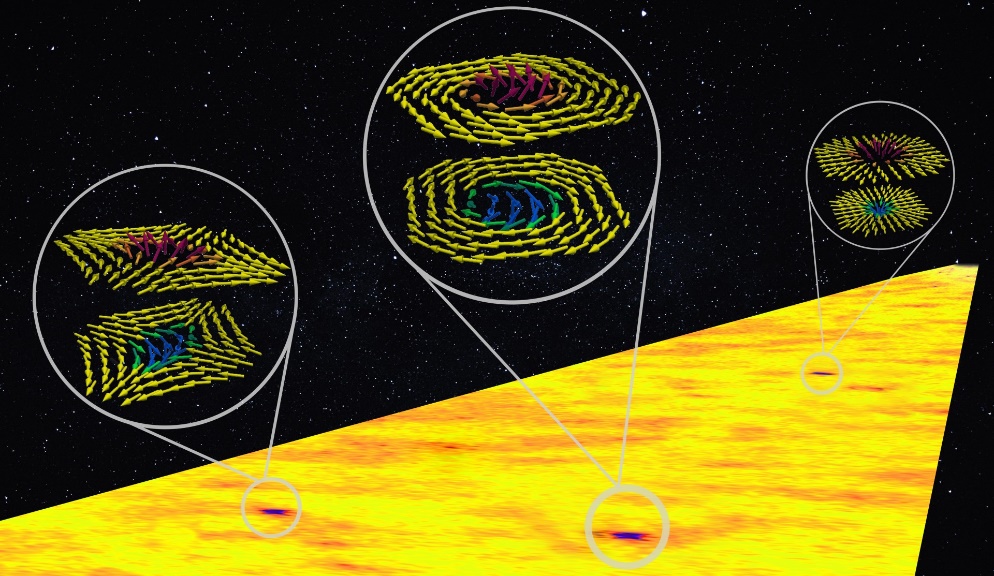
Motivation: Today's computing ecosystem uses 10% of global electricity and contributes 2% of emissions (at par with aviation!). With the rise of AI, autonomous devices and big data, this energy demand will increase sharply, putting strain on global resources. Heart of the problem is that charge-based silicon platforms are volatile, inefficient and serial. This has resulted in a quest for novel quantum materials hosting rich emergent physical properties that can be harnessed to yield high-speed and energy-efficient functionalities.
Topologically-rich antiferromagnets (AFMs), consisting of vortex-like whirling spin textures, are especially promising in this regard, as they host a robust non-volatile magnetic order. It has been theoretically predicted that such nano-whirls can move at incredible speeds of kilometres/second and host rich dynamics up to terahertz frequencies, i.e. 100-1000 times faster than conventional silicon devices. Such magnetic ‘nano-whirls’ could be used to power reservoir computing or logic-in-memory, enabling next-gen AI hardware [1].
We have pioneered the discovery and control of AFM solitons at room temperature, thus, experimentally opening the field of topological antiferromagnetism [2,3,4,5,6]. Our breakthroughs have been published in Nature (2021), Nature Materials (2018), Nature Materials (2024a), Nature Materials (2024b), Nature Communications (2021) etc.
Project Scope: The goal of these DPhil projects is to push this young field toward the ultra-fast dynamical frontiers and thus open doors to its practical utilisation. Students will learn to use cutting-edge spectro-microscopy and dynamical imaging techniques to explore fast AFM dynamics. They will pioneer a versatile toolbox – involving quantum materials design, spintronic control, combined with electrical and optical excitation – to enable targeted spatio-temporal control of AFM nano-whirls. These fundamental breakthroughs are urgently needed to enable transformative next-generation applications, like terahertz nano-oscillators, AFM magnon nano-emitters and terahertz reservoir computing to develop next-gen AI.
We are looking for passionate physics students with:
- a strong academic track record and good exposure to hands-on research
- experience in coding or simulations (e.g. using Python, MATLAB etc.)
- a keen interest in both experimental research and computational modelling
Scientific Techniques: Successful candidates will develop research expertise in both fundamental and applied research. Depending on the candidate's interests, the project may involve the development of one or more of the following techniques in combination with computational modelling.
Quantum materials design: Growth of high-quality crystalline thin films and free-standing nano-membranes [2-5] using integrated pulsed laser deposition and UHV sputtering at advanced growth suites in Oxford Physics, as well as at the Albert Fert Laboratory - CNRS/Thales (Paris) and National University of Singapore (Singapore).
Device fab and characterisation: Nanofabrication of prototype devices will be done using e-beam/optical lithography, laser writing and focused ion beam milling in Oxford’s Class 100 and 1000 cleanrooms. Structure/surface characterisation will be done using in-house high-resolution diffraction, reflectometry and scanning probe microscopy. Physical properties measurement system and custom-built equipment will be used to study electrical properties.
Advanced Microscopy: Imaging of nanoscale multi-functional domains, which form the fundamental unit of information storage in oxides, will be done using X-ray spectro-microscopy techniques including X-PEEM [2,6], STXM [3], holography [7] and ptychography at leading synchrotrons - Diamond (UK), PSI (Switzerland), Soleil (France) etc. We also employ quantum NV-magnetometry, in collaboration with the Mete Atature group (Cambridge), to perform stray field imaging [4].
Ultra-fast Dynamical Imaging: Time-resolved nanoscale dynamics will be captured using cutting-edge pump-probe X-ray imaging at synchrotrons – BESSY (Germany), PETRA (Germany) and Free Electron Lasers – FERMI (Italy), SwissFEL (Switzerland). Frequency-resolved dynamics will be captured with pump-probe micro-focus Brillouin Light Scattering.
Simulations and Theory: Dynamical evolution of magnetic states will be modelled using Oxford’s high-performance computing service (ARC). We use both atomistic and micromagnetic software [8]. Students will also collaborate with the leading theory expert Olena Gomonay (Mainz) to develop analytical solutions.
Students will have opportunities for extended stays in world-leading groups of our collaborators - Manuel Bibes (France), Dirk Grundler (Switzerland) and Ariando (Singapore).
If you want to join our team, contact Hariom Jani (hariom.jani@physics.ox.ac.uk) with your CV and a statement of interest.
Deadline: 8 Jan 2025
Apply here: https://www.ox.ac.uk/admissions/graduate/courses/dphil-condensed-matter-physics
References:
[1] J Grollier et al. Nature Electronics 3, 360 (2020).
[2] H Jani et al. Nature 590, 74 (2021).
[3] H Jani,* J Harrison* et al. Nature Materials 23, 619 (2024).
[4] A Tan,* H Jani,* et al. Nature Materials23, 205 (2024).
[5] H Jani, et al. Nature Communications 12, 1668 (2021).
[6] F Chmiel et al. Nature Materials 17, 581 (2018).
[7] J Harrison, H Jani et al. Optics Express 32, 5885 (2024).
[8] J Harrison, H Jani et al. Physical Review B 105, 224424 (2022).
[9] ZS Lim,* H Jani* et al. MRS Bulletin 46, 1053 (2021).