Fast Gates
Ion trap quantum computers utilise the electronic and motional states of ions to prepare, control and read information. Ions are perfectly indistinguishable qubits, have unrivalled coherence times and are relatively easy to manipulate through the strong coupling that is observed between external electromagnetic fields and their internal electronic states. They have proven to be amongst the strongest candidates for a quantum computing platform.
Up until 2018, the slow speeds attained for two-qubit gates performed in ion traps threatened to become a bottleneck for quantum computing on this platform. Entangling gates had been limited to the adiabatic regime, unable to exceed the MHz motional frequencies of the ions. Although our group demonstrated that this limit could be experimentally surpassed [Nature 555, 75–78], gates performed in times comparable to the ones observed in solid state qubits suffer from large error rates, falling short of the fidelity required for fault-tolerant quantum computing.
The speed limitation can be understood through exploration of the mechanisms behind two-qubit gates. In ion traps, qubit states are most commonly driven through Raman transitions, involving a third auxiliary short-lived (~ns) state and hence suffering from off-resonant photon scattering. This error becomes more prominent with increasing gate speed, due to the respective required increase in optical power. Alternatively, an ionic qubit can be driven directly on a long-lived (~s) quadrupole transition. In this case, a faster gate will be less sensitive to scattering errors, due to the relatively long lifetime of the decaying state. Nonetheless, quadrupole gates achieve lower fidelity than their Raman counterparts as they are extremely sensitive to laser frequency noise, making them less experimentally desirable.
In the Fast Gates project, we aim towards high-fidelity, high-speed entangling gates by operating in the far-out-of-adiabatic regime. Here, all motional modes of the ion crystal couple significantly during the interaction; this large Hilbert-space can be tamed by employing amplitude pulse-shaping techniques [NJP, 16(5), 53049].
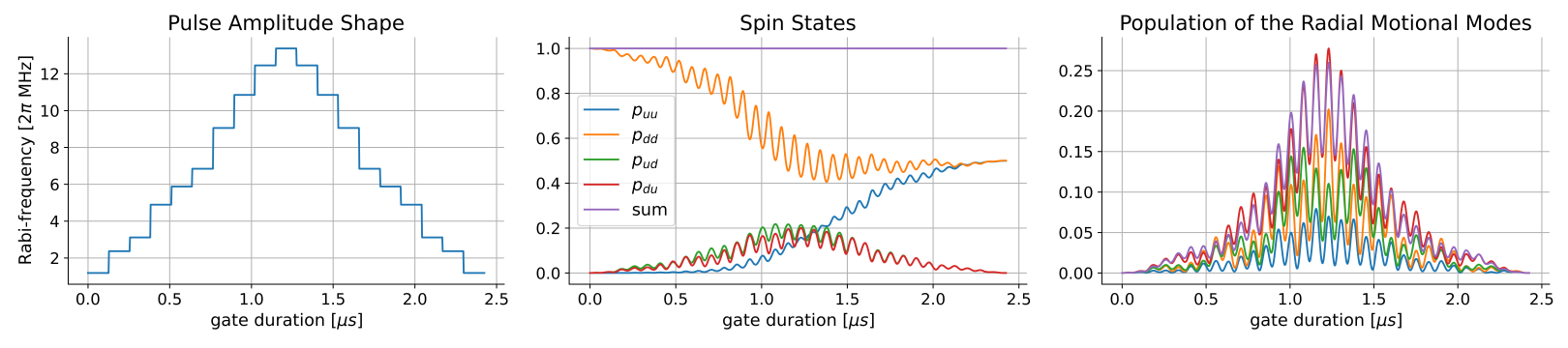
The non-adiabatic regime is rich in novel physics. For example, as the gate time becomes comparable to the timescale of the mediating ion crystal we can study the origin of entanglement creation [Phys. Rev. Lett. 94(5), 50504].
To generate the extreme light intensities needed for these operations and for the many interesting applications of these protocols, we require single-ion addressing and a powerful laser driving our quadrupole transition. We have begun the process of designing, planning and most recently building a novel trap setup for 40Ca+ ions, incorporating these features. The optical addressing will be chosen such that we can implement a novel and relatively unexplored ion-light interaction dynamic. Through this interaction we are not only able to cancel the most prominent and restrictive noise present in our entangling gate scheme, but we can also explore the interaction itself. We anticipate promising applications in quantum sensing [Phys. Rev. A, 101(5), 52331] and adiabatic quantum simulation [NJP, 12(9), 95019].
People
Current
- Dr. Chris Ballance (PI)
- Oana Bazavan (Postdoc)
- Sebastian Saner (Postdoc)
- Donovan Webb (Graduate student)
- Dr. Kai Shinbrough (Postdoc)
- Iver Oevergaard (Graduate student)
Former
- Dr. Mariella Minder (Postdoc)
- Dr. Amy Hughes (Postdoc)
- Dr. Vera Schäfer (Graduate student)
Contact
Publication highlights
- Fast quantum logic gates with trapped-ion qubits, Nature 555, 75–78 (2018)