Quantum Networking
We operate an elementary quantum network of two trapped-ion nodes connected by an optical fibre link to explore applications of remote entanglement on distributed quantum computing, metrology and fundamental physics.
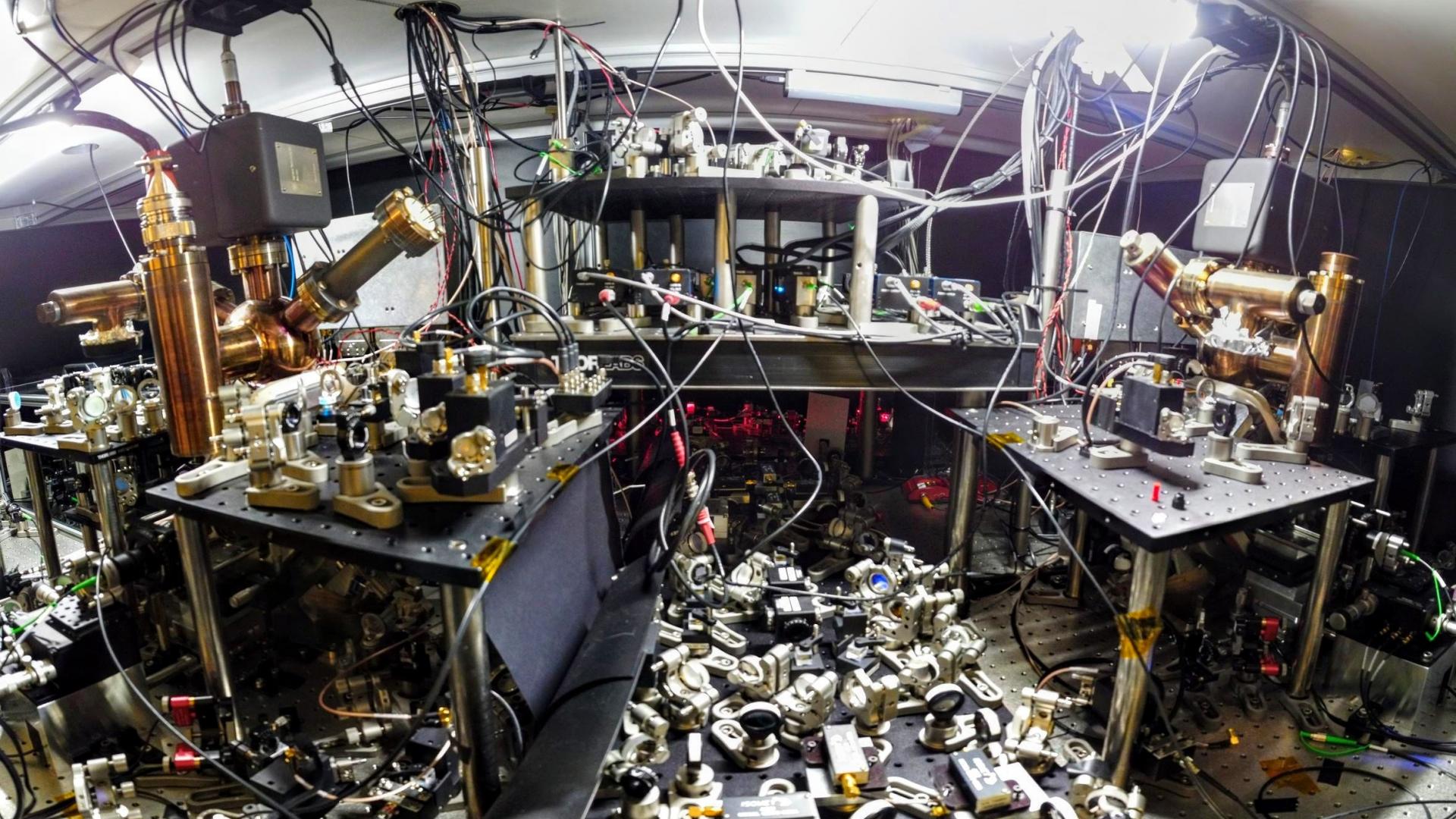
Alice and Bob
Trapped ions and photons: complementary quantum systems
Both trapped ions and photons – particles of light – behave in accordance with the rules of quantum mechanics, and can be manipulated with exquisite precision using room-temperature technology, making them interesting for quantum information processing. In some aspects, they are diametrically opposite qubit technologies: Trapped ions are kept virtually at standstill in ultra-high vacuum systems, where they are routinely laser-cooled to just above absolute zero, and multiple trapped-ion qubits are readily made to interact using their electric repulsion (enabling the record-precision quantum logic gates demonstrated here in Oxford and elsewhere). Photons, on the other hand, naturally propagate at the speed of light and barely interact with each other or other matter, allowing single photons to travel over many kilometers of optical fibre without losing their quantum information.
This duality makes hybrid systems consisting of both trapped-ion and photonic qubits a fertile ground for scientific exploration as well as for technological applications. In particular, a key motivation behind our work is a proposal for a scalable quantum information processing architecture, where – in much the same fashion as how today’s supercomputers are built from racks upon racks of smaller servers – a number of relatively simple ion-trap processing nodes are banded together into one big computer using optical fibre links.
Alice and Bob
In our laboratory, we operate an elementary version of such a quantum network: two dual-species ion trap nodes, 'Alice' and 'Bob', linked by a few metres of optical fibre. At the heart of each trap is a surface-electrode trap chip, which allows us to trap multiple chains of ions that can be independently moved around, split up further or recombined to implement complex algorithms. We use two different ion species chosen to excel in different functions: 43Ca+ ions serve as our 'application' qubits, offering long-lived storage and precise manipulation using the well-established, laser-based techniques previously developed in our group (and elsewhere). 88Sr+ ions serve as our 'interface' qubits for entanglement generation.
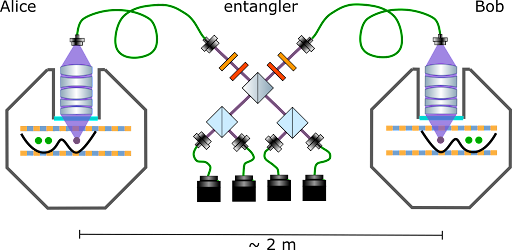
separate segmented Paul traps. Photons emitted by the Strontium ions are
collected using high numerical aperture objectives and used to generate remote
entanglement between the ions. Calcium ions are used as memory and
processing qubits.
To establish entanglement, picosecond-scale synchronised laser pulses excite an ion in each trap to a short-lived state from which there are multiple possible spontaneous decay paths, leading to entanglement between the ion’s resulting state and the polarisation of the emitted decay photon. The photons are coupled into fibres using lens objectives and interfered in the 'Entangler' beam-splitter network, which has single-photon detectors at its output. The observation of coincident detector clicks heralds the successful entanglement swapping process – starting from two pairs of ion-photon entanglement, the photons have been destroyed, leaving the ions in an entangled state instead. Using mixed-species quantum logic gates, the entanglement can then be transferred onto 43Ca+ ions for further storage and use.
Research directions
As a first milestone, we were able to improve on the state-of-the art in remote entanglement generation [Phys Rev. Lett. 124, 110501], showing both a high fidelity of 96.0(1)% (i.e. a low error rate) and a high generation rate of more than hundred Bell pairs per second between Alice and Bob. After recent automation improvements, the system can operate at this performance level for days, with the two nodes autonomously coordinating calibration routines as necessary.
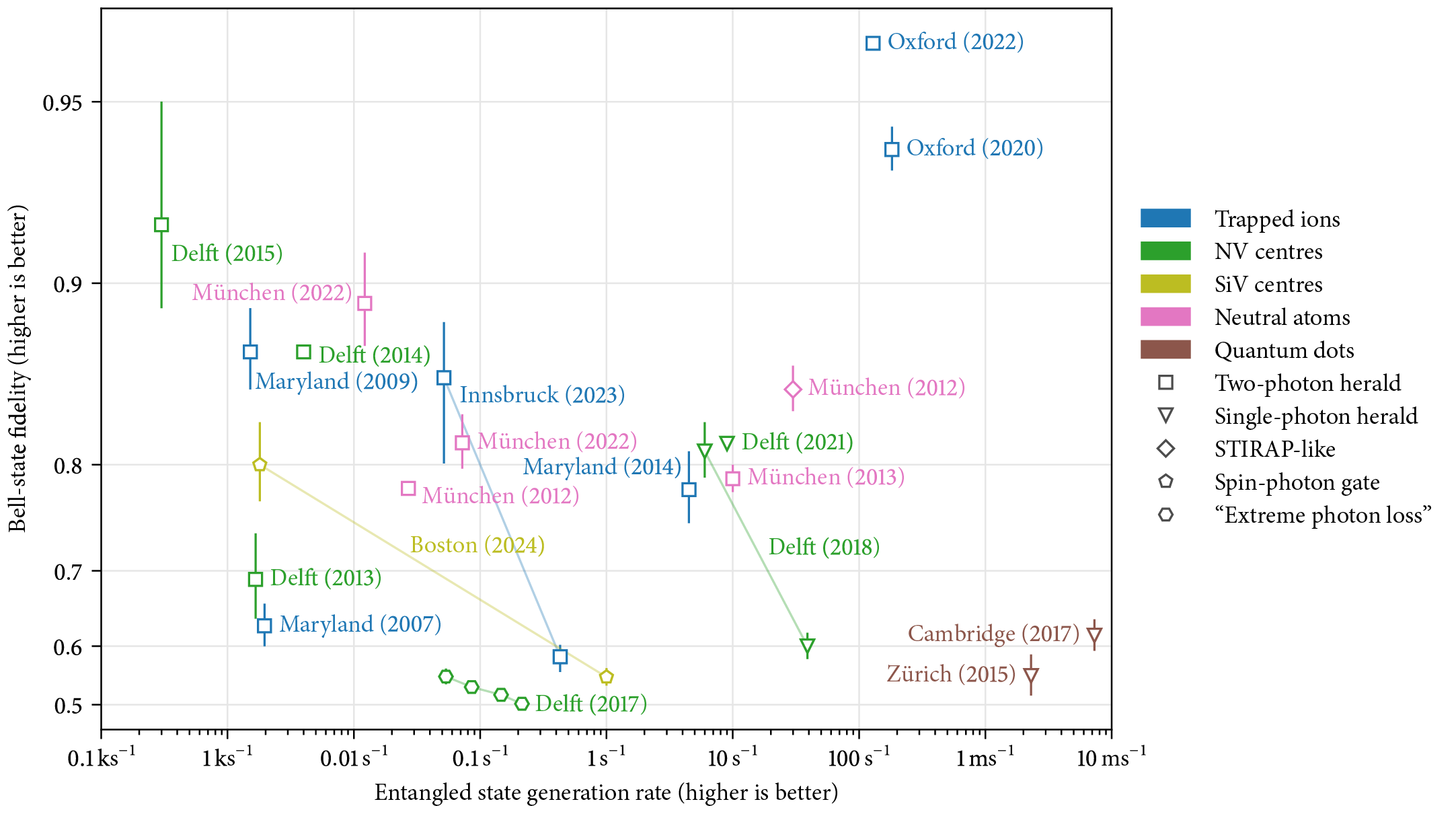
Building on this, we are now investigating a number of more complex protocols and applications. From a quantum computing perspective, even our state-of-the-art error rates still exceed that of local entangling gates by far, making entanglement distillation, where multiple raw Bell pairs are combined into a single higher-quality one, an obvious next step. High-fidelity remote entanglement is a rich resource for many other applications as well. We are currently pursuing a number of projects in client-server quantum computing, quantum cryptography, entanglement-enhanced metrology and quantum foundations.
People
Current
- Prof. David M. Lucas (PI)
- Dr. Gabriel Araneda (Senior Postdoc)
- Dr. Raghavendra Srinivas (EPSRC Research Fellow)
- Dr. David P. Nadlinger (Junior Research Fellow, Merton College)
- Dr. Peter M.L. Drmota (Postdoc)
- Dr Jake Blackmore (Postdoc)
- Dougal J. Main (Graduate student)
- Ellis M. Ainley (Graduate student)
- Ayush Agrawal (Graduate student)
- Adam Martinez (Graduate student)
Former
- Dr. Bethan C. Nichol (Postdoc)
- Dr. Chris J. Ballance (PI)
- Dr. Joseph Goodwin (Postdoc)
- Dr. Laurent Stephenson (Graduate student)
- Dr. Shuoming An (Postdoc)
- Dr. Tim Ballance (Postdoc)
Publication highlights
- Distributed Quantum Computing across an Optical Network Link (preprint), arXiv:2407.00835
- Multipartite Entanglement for Multi-node Quantum Networks (preprint), arXiv:2408.00149
- Verifiable blind quantum computing with trapped ions and single photons,
Physical Review Letters 132, 150604 (2024), arXiv:2305.02936
- Robust Quantum Memory in a Trapped-Ion Quantum Network Node,
Physical Review Letters 130, 090803 (2023); arXiv:2210.11447
- An elementary quantum network of entangled optical atomic clocks,
Nature 609, 689–694 (2022); arXiv:2111.10336
- Experimental quantum key distribution certified by Bell's theorem,
Nature 607, 682-686 (2022); arXiv:2109.14600
- Micromotion minimisation by synchronous detection of parametrically excited motion (preprint);
arXiv:2107.00056
- High-rate, high-fidelity entanglement of qubits across an elementary quantum network,
Physical Review Letters 124, 110501 (2020); arXiv:1911.10841