24 May 2021
Drive smarter, not harder: low convergence ratio ICF experiments may still yield substantial gains
A simulation campaign shows that nuclear fuel compressed to comparatively low densities in inertial confinement fusion experiments can still generate useful amounts of energy.
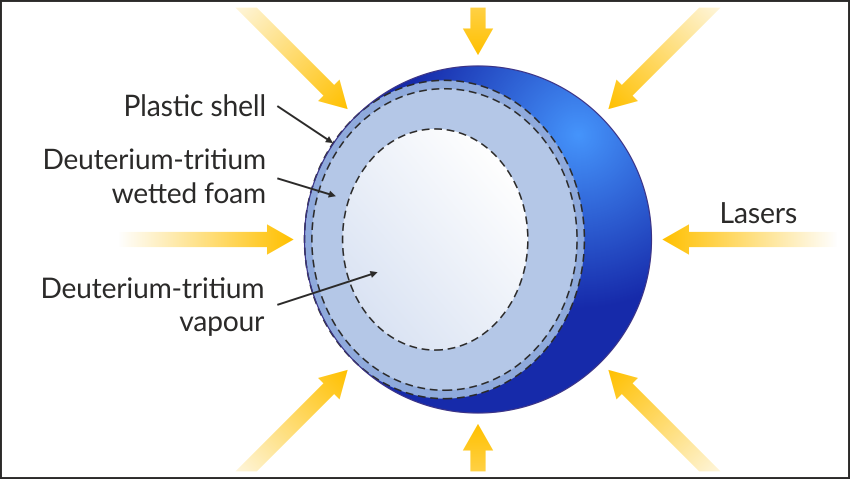
The task of bringing fusion power to Earth remains one of the most formidable scientific and technological challenges of our time. One promising means of producing controlled nuclear fusion reactions in the laboratory is direct-drive inertial confinement fusion (ICF), in which a small, spherical pellet of deuterium-tritium fuel is fired upon from all directions simultaneously by an array of converging high-power laser beams. The resulting implosion compresses and heats the fuel to such a degree that a fleeting nuclear fusion event is triggered, releasing energy in the form of neutrons that can be collected and harnessed.
The conventional goal of ICF experiments is to compress the fuel as much as possible, in order to maximise the energy yielded by the fusion reaction. However, the greater the target’s final compression (characterised by its so-called convergence ratio), the more vulnerable it is to hydrodynamic instabilities. Such instabilities cause initial imperfections in the spherical capsule’s symmetry to grow exponentially with time, compromising the integrity of its layered structure and ultimately reducing the energy that it releases.
Robert Paddock and co-authors suggest an alternative approach to ICF. They advocate deliberately applying less compression to the fuel capsule so as to suppress the instabilities that hamper the implosion process. By analysing a set of over ten thousand simulations of ICF implosions, they were able to demonstrate that, even in this low convergence ratio regime, it is still possible to generate relatively large amounts of fusion energy.
"We showed that a gain of 50 – which is what you would likely need for an economically viable fusion reactor – can be achieved using this technique alone, for an input energy of around 8.5 megajoules (MJ)," said Robert.
While 8.5 MJ exceeds what can be delivered by current facilities, impressive gains were also predicted at the kind of input energies achievable today at Laser Mégajoule and the National Ignition Facility (NIF):
"We predicted gains of 0.2 for around 0.8 MJ, and 0.8 for 1.7 MJ. These, if achieved experimentally, would represent record gains for these energy scales, showing clearly the promise of this technique."
Since lower convergence-ratio compression results in a much more symmetric implosion, the authors are also able to model it reasonably confidently using relatively simple one-dimensional hydrodynamics codes like Hyades:
"These codes are very fast and easy to work with compared to 2D/3D codes, and so it is much easier to rapidly explore a parameter space to find the best performance."
The authors plan to expand their simulation campaign to include two- and three-dimensional simulations of particularly promising targets, in order to confirm that instabilities really are suppressed in this low convergence-ratio regime. In the longer term, the authors also intend to conduct experiments to verify the predictions of their simulations, and thus demonstrate conclusively the merit of their approach. Characterisation of the targets to be used in these experiments will be aided by an upcoming equation-of-state experiment at the CLF Vulcan laser facility, which the authors will undertake in 2022.
Full article: One-dimensional hydrodynamic simulations of low convergence ratio direct-drive inertial confinement fusion implosions by R. W. Paddock, H. Martin, R. T. Ruskov, R. H. H. Scott, W. Garbett, B. M. Haines, A. B. Zylstra, R. Aboushelbaya, M. W. Mayr, B. T. Spiers, R. H. W. Wang, and P. A. Norreys, Phil. Trans. R. Soc. A 379: 20200224.
Return to HEDLines