11 January 2022
Links in another world: the influence of a shattered crystal on the world's most expensive thermometer
A computational study outlines the physical limits and requirements of a dynamic temperature diagnostic for dynamic-compression experiments.
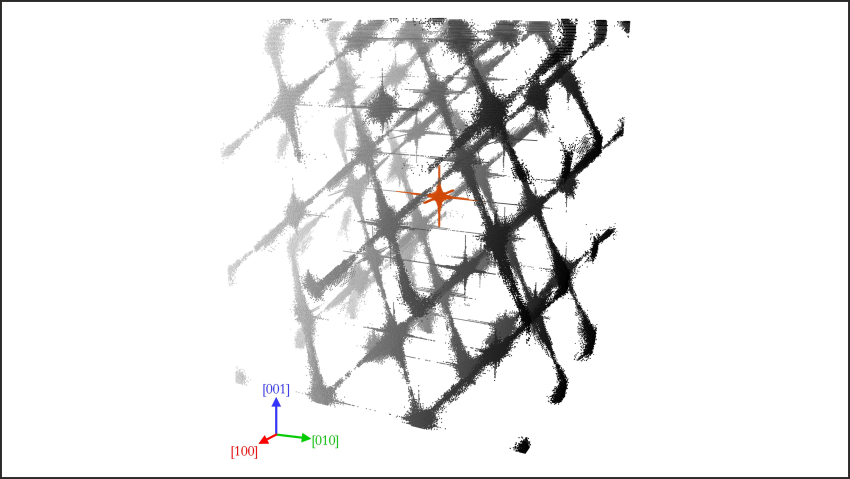
The field of high-energy-density (HED) physics investigates matter in extreme conditions, such as ultrahigh pressures and temperatures. Such states are scarce in everyday life, but can be obtained for fractions of a second through shocking material with intense laser pulses. Shock physics in particular allows mankind to investigate the most fundamental properties of HED matter and enables us to make predictions about the properties of the rich and ever-growing set of exoplanets we've discovered within our universe.
These delicate measurements can be experimentally challenging due to their short life (a few nanoseconds at most) and their intrinsically chaotic nature. Hence, high-pressure research greatly benefits from ultrafast diagnostics that can measure the physical properties of matter under compression. Whilst there are numerous methods of determining the absolute temperature of matter (e.g., the thermal expansion of a well-characterised fluid), HED research lacks a reliable dynamic temperature diagnostic.
One promising solution is the usage of inelastic x-ray scattering (IXS) and the principle of detailed balance, which is routinely done at static synchrotron experiments. It disperses a scattered x-ray photon in energy, generating two spectral peaks related to the creation of a phonon and the absorption of a pre-existent one. Based on their relative intensity, the absolute temperature of the scattering sample can be calculated. Due to phonons being involved, this method requires extremely high energy resolution (tens of milli-electronvolts) and a high incident photon count (the IXS cross-section is much lower than that of Bragg diffraction).
To extend this method to shock experiments, an extremely intense x-ray source is required since the short dynamic timescale implies a short photon-collection time. This is where x-ray free electron lasers (XFELs) come into play: multi-billion-dollar facilities like the EuropeanXFEL (Germany) or SLAC (USA) that are capable of delivering pulsed x-rays of extreme brilliance and sub-100-femtosecond duration.
With the aid of these extreme sources, successful static proof-of-principle measurements have been achieved for well-behaved samples, specifically a near-perfect crystal of diamond. Shocks, however, induce plasticity (chaotic, irreversible displacement of atoms), which can impair the IXS signal as Oliver Karnbach et. al. have shown.
The phonons used to determine the sample temperature live in reciprocal space (the space that describes which oscillation modes may exist within the sample) between Bragg spots. The latter are its primary modes and are well-defined, localised peaks for perfect crystals. However, a crystal usually shatters during shock, which can cause linear streaks to arise (see image above), that connect a subset of the Bragg spots.
The phonons between such linked spots give rise to a third spectral peak: quasi-elastic scattering. This physics, which arises via the finite size of the crystallites, affects experiments, but in the future can be mitigated by better resolution at even brighter sources, such as at the HED endstation of the EuropeanXFEL operating at full capacity.
Ultimately, IXS is an extremely useful diagnostic for dynamic HED research that will improve our understanding of the fundamentals of worlds beyond ambient pressure and temperature.
Full article: Molecular dynamics simulations of inelastic x-ray scattering from shocked copper by O. Karnbach, P. G. Heighway, D. McGonegle, R. E. Rudd, G. Gregori, and J. S. Wark, Journal of Applied Physics 130: 125901 (2021).
Return to HEDLines